Thinking
Inside the Box
>>
Simulating
the universe's 13 billion years sheds light into the heart of
its darkness.
The
movie doesn't take long -- 49 seconds, in fact. Yet in that short
time all of existence unfolds on the computer screen: sky-blue
pinpricks morph into brilliant blue filaments, glowing in a black
expanse and contained, very neatly-and completely outside the
realm of reality-within the three-dimensional parameters of a
box.
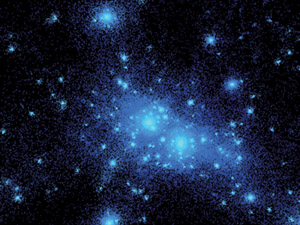
VIEW THE MOVIES
|
13
billion years
5.0 mb, 21 sec.
The
Magazine has reduced the size of Andrey Kravtsov's
movies. Play times vary according to Internet connection
speeds. To save, PC users may right click here for
movie
1
or movie
2. To view the videos, you must have
the QuickTime player installed. A free download
is available on Apple's
website.
|
|
The
movie ends and then begins again, silently looping as Andrey Kravtsov,
assistant professor of astronomy and astrophysics, explains what
he's created: a high-resolution, fast-forward simulation of the
entire 13 billion years of the universe-well, minus the first
100 million years, since the universe's earliest evolution is
simple enough to be modeled on paper with linear equations, so
there's no sense in devoting computational resources to simulating
it. (The incandescent blue, on the other hand, seems to scoff
at the observation reported this March that the universe is not
even a pale turquoise but rather a go-with-everything beige.)
Like
any good scholar, the 29-year-old Kravtsov begins by defining
his terms. Pointing to a Z in the screen's upper left-hand corner,
he explains with his Ukrainian accent, "Z is the redshift,
a measure of time in astronomy." Because the speed of light
is finite, the light now visible from galaxies was emitted long
ago. And because the universe is expanding, this light is redshifted,
meaning its wavelengths have shifted to longer, lower-energy,
"redder" wavelengths. The greater the redshift, the
further back in time the object we "see" existed. Kravtsov's
simulation begins with 2 million particles at a redshift of about
30, or about when linear models begin breaking down, and ends
at Z = 0. In lay terms, that's present day.
The
catch is that Kravtsov's pinpricks and filaments can't, in fact,
be "seen" at all. They represent nonbaryonic particles:
dark matter. Which explains Kravtsov's color choice: the blue
represents the "coldness" of dark matter. Unlike ordinary
visible matter, dark matter is not made up of protons or neutrons
and is thought to interact with ordinary matter only gravitationally.
The absence of electromagnetic interaction between dark matter
and baryons means that it does not glow the way that quasars,
stars, supernovae-even people and trees, though in infrared-do.
Astronomers now believe that nonbaryonic matter accounts for 85
percent of the matter in the universe. "So the evolution
of the universe is actually dictated by dark matter," says
Kravtsov. "We-the baryonic matter-just follow along."
His
movie, one realizes, portrays the universe in the nude: what we'd
see if we could don X-ray-er, enlightened-matter goggles-and look
beyond the universe's flimsy baryonic clothes to watch the dark
flexing of the powerful limbs and muscles beneath. And that's
why Kravtsov's work is important: after he's written the equations
and crunched the numbers, the simulated dark matter progresses
along the redshift, with particles gravitating toward each other
in ever increasing numbers, first creating galaxies and then clusters
of galaxies and finally ending up in a configuration that remarkably
resembles exactly where we-the baryonic matter-are today.
The
scheme "works because it accounts for the structures we can
see," says Bruce Winstein, the Samuel K. Allison distinguished
service professor in physics, the Enrico Fermi Institute, and
the College. "Dark matter, dark energy-we can't see these
things in the lab or in the telescope, and yet they've become
our two fundamental pursuits. So lacking the ability to observe
them, we simulate them.
"This
simulation," he continues, "wouldn't work without those
two things in there. If we can figure out how the current structure
came about, we can see what's moved it there. If the simulation
had too much dark matter and dark energy, it wouldn't look the
same."
Winstein,
a particle-physicist-turned-astrophysicist, directs the seven-month-old
Center for Cosmological Physics (CfCP), for which Kravtsov is
among six new faculty recruits. (He comes from a Hubble fellowship
at Ohio State University after earning a Ph.D. in astronomy and
computer science at New Mexico State University in 1999.) Launched
with a five-year, $15 million grant from the National Science
Foundation, the CfCP is, according to its Web page, "devoted
to exploiting the connections between physics at the smallest
scale-interactions of the quarks and leptons-and at the largest
scale-the constitution and birth of the cosmos itself."
Kravtsov's
work marries the quests to understand the quark and the cosmos
quite nicely. For the vast majority of the simulation, from Z
= 30 to Z = 10, the matter seems to be distributed uniformly.
But at the tiniest level there are irregularities, "primordial
quantum perturbations" left over from the Big Bang that cause
some particles to be ever so slightly closer to each other. These
perturbations, says Kravtsov, are the "seeds of the structures"
we see today.
"Gravity,"
he explains, "tends to make perturbations grow. As the density
of matter in a perturbation increases, so does gravitational pull.
Gravity thus leads to instability, and instability leads to growth
of perturbations and collapse." That is how structures in
the universe-filaments, clusters, galaxies, stars-form. And the
collapse proceeds even as the universe expands. Moreover, it was
recently discovered, the expansion is currently accelerating due
to the presence of mysterious "dark energy," which dominates
the energy density of the universe.
The
trick to Kravtsov's simulation is that the box and the matter
in it are "co-moving," or expanding with the universe
so that the box appears to be the same size even as the universe
expands. Kravtsov's simulation has one of the highest dynamic
ranges among simulations of its kind-meaning that it's highly
pixilated and therefore highly detailed, but only in the regions
of interest: areas such as clusters and galaxies dense with matter.
"The
question for us is always, What is the smallest scale you can
follow compared to the size of the box?" he says. "In
theory we would like to be able to push the algorithms to simulate
the formation of stars." In reality, he's been able to "reach
in" below a kiloparsec, or about 3,270 light years, roughly
one-thirtieth the size of the Milky Way. The technique, called
adaptive mesh refinement, is a trick engineers-specifically airplane
designers-have used for 20 years, but it's relatively new to astrophysics.
After
a year of creating the simulation's numerical code, Kravtsov spent
a week running the code at the National Center for Supercomputer
Applications in Urbana-Champaign, Illinois, churning the algorithms
on 16 processors. The simulation's output was then compared against
observed structures. His slimmed-down version is a 45-megabyte
movie that runs on Windows Media Player.
In
his office on Chicago's campus, Kravtsov double-clicks another
movie file. The box rotates as the particles float toward one
another, revealing from all angles the delicate beauty of the
universe's filamentary structure. It looks exactly like the pulsating
and branching blood vessels of the human body. "They actually
have a lot in common," he agrees, "because they're both
approximately fractal structures."
The
structures freeze, and the question is itching to be asked: Well?
What happens next? Will gravity keep pulling the Milky Way and
Andromeda toward one another, as it has since Z = 3, until they
collide-and if so, when will that happen? And when the box has
gotten so big that nothing's close enough for gravity to pull
it, what will happen then?
Kravtsov
gives the pragmatic shrug of an experimental scientist: "Yeah,
probably in 6 billion years they'll collapse. The simulation is
designed for comparisons with actual observations, so to run it
further would be a waste of computational resources."
He
clearly doesn't see why you'd want to anyway-there's still too
much to learn inside the box to think about straining its corners
just yet.
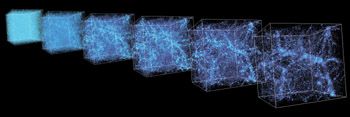
1.
The simulation begins where linear equations break down-about
13 billion years ago. Algorithms based on the initial conditions
predicted by inflationary models of the universe reveal what appears
to be a uniform blanket of dark matter.
2.
That which appears uniform is not: there are small fluctuations
in the density of matter created by a quantum stir billions of
years ago. In a snowball effect, the fluctuations grow in size
by many orders of magnitude during the inflationary expansion
of the universe.
3.
Galaxy distribution is strongly "biased": galaxies tend
to form in clusters rather than forming uniformly across space.
4.
The evolution of the cosmic structure is hierarchical: gravitational
collapse first builds small objects-galaxies-and then larger structures-clusters
of galaxies. Clusters tend to move toward each other and form
filamentary structures, flat and elongated.
5.
Voids develop between intersecting filaments, and clusters grow
larger. Galaxy clusters are the most massive self-gravitating
objects in the universe and therefore provide the most clues about
its age, size, and ultimate fate.
6.
The "present day" distribution of dark matter: if the
nonbaryonic matter in the final frame were "dressed"
with ordinary matter, this image would look very much like the
distribution of galaxies and galaxy clusters observed in the real
universe.