More Than Meets the Eye
By Megan Lisagor
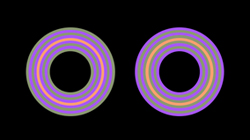 |
The
perceived color of the two orange rings varies—but they’re
identical, printed in the same ink. The patterned background
of purple and lime circles causes the illusion, a phenomenon
known as chromatic induction. |
Although the eye is vision’s front
door, the brain creates perception. Researchers want to reveal how
the mind translates light into visual experience
White boards, splattered
with red, green, and blue equations, cover the walls. Computers
crowd paper-stacked desks. Programming manuals line bookshelves.
In offices that resemble the habitats of their mathematics or computer-science
colleagues, neurobiologists, ophthalmologists, and psychologists
investigating visual perception wield mathematical and computational
models not to describe the physical world, but rather to decipher
how it’s seen.
In laboratories spread across campus, they have
projects under way to unlock the inner workings of the relationship
between the eyes, brain, and perception—the process of interpreting
sensory information. Exploring both high- and low-level functions,
from memory to motion, their studies may even lead to a cure for
blindness. “The visual system is not at all like a camera,”
says Steven Shevell, a professor in psychology and ophthalmology
& visual science, dispelling a popular analogy. Going far beyond
what a camera does, the visual system “represents objects
in meaning.”
The system activates when light waves reflect
off objects and enter the eye. Passing through the cornea, light
reaches the retina, where rods and cones convert it into electrical
impulses. Those impulses travel along the optic nerve, a mass of
ganglion cells, to the back of the brain and the primary visual
cortex—perception’s powerhouse. From there, messages
circulate to other regions and, presto, a three-dimensional, colorful
mental image surfaces.
Scientists trying to break down the events that
lead to visual experience have a lot of ground to cover. “Just
seeing an object, for example, causes many thousands of cortical
neurons to become active,” Chicago neurophysiologist David
Bradley notes, “and their activities together create and shape
what we perceive. But how are these activities understood, or ‘read
out’ by the brain? If we could actually measure all these
activities, would we know what perception was?”
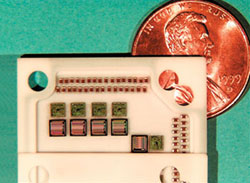 |
Researchers
want to restore sight to the blind by creating a device that
triggers the brain with electrical pulses. They’ve developed
an implantable stimulator, the size of a coin. |
Seeing is believing, right?”
jokes Shevell, 54. Not exactly: “We do not have an accurate
representation of physical reality,” he explains. The properties
that make up what people “see”—color, orientation,
texture, depth, motion, size, location—are at the mercy of
both the eyes and the brain. “The brain,” for its part,
“makes an educated guess,” he says. “The driving
force behind our research is trying to understand how our natural
world is represented perceptually.” For starters, color—his
area of expertise—is a mental, not physical, phenomenon.
To illustrate his point, Shevell, dressed in
basic black, offers a quick neighborhood tour on a July-hot April
day. “Sometimes it just strikes me,” he says, turning
toward an evergreen on 57th Street outside Snell-Hitchcock Halls.
“You think that direct sunlight is shining” on the shrub’s
tips, but it’s “the lighter color of new buds. The actual
color we perceive—either a different color at the tips or
the whole plant as one color—is a mental process in which
the visual system interprets the light.”
Shevell, no one-trick pony, continues the tour.
Fishing a white plastic bag out of a nearby trash can, he holds
it behind a bunch of pale yellow daffodils, and suddenly the flowers
seem more yellow. The eye and brain sharpen the perceived difference,
he explains, making the color stand out.
Such sharpening, he says, “allows you to
see differences among things that are close by.” For instance,
the contrast between dark ruby cherries and their yellowish counterparts
helps pickers discern good fruit from bad. Landscapers also play
off the phenomenon—say by putting pale pink flowers next to
white ones—so certain colors pop. “Monet understood,”
Shevell notes, and “he painted his garden at Giverny in contrasting
hues.”
The brain’s control over what people see
has commercial applications too. Grocery-store owners often install
track lighting above apples to create the illusion of a redder fruit,
Shevell says, and restaurateurs know that stationing a tungsten
lamp over carved roast beef produces a “nice, red, and juicy”
look. To unriddle those gimmicks, he harks back to high-school physics,
dismantling ROY G BIV, the mnemonic device to learn the color spectrum
(red-orange-yellow-green-blue-indigo-violet). Humans only “perceive
light that way,” he says. In fact, “light has a wavelength,
not a hue,” so color represents the brain’s responses
to light of varying wavelengths.
Further, like in the daffodil example, an object
can look different depending on its context, a phenomenon known
as chromatic induction. “The neural representation of a particular
area [of a scene] is influenced not only by the light in that area,”
Shevell says, “but also by the light throughout the scene.”
Back in his Biopsychological Research Building office, he whips
out another illustration: a white piece of paper with four rings
printed on a background of purple and lime circles. The rings appear
multiple shades of orange, but they’re actually identical,
the same ink.
To account for the false impression, Shevell
and Florida Atlantic University’s Patrick Monnier theorized
that a particular type of neuron in the primary visual cortex—responding
exclusively to impulses from the retina’s short-wavelength
cones—causes shifts in color appearance with patterned backgrounds
composed of two chromaticities. “We’ve revealed a new
kind of neuron in the brain,” he says, “and now that
we understand the way it works we know it can lead to remarkably
big errors,” as with the rings. After they published their
theory in the August 2003 Nature Neuroscience, neuroscientists
reported finding a similar cell in macaque monkeys.
“Vision is so automatic to us,” Shevell
says, offering up a modern analogy: “When you push the buttons
on your cell phone, you don’t think about the technology that
makes it work.”
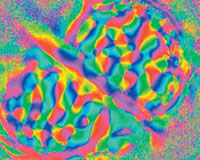
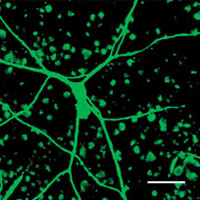
|
Brain,
eye, memory: to see how visual information gets represented,
neurobiologist Naoum Issa mapped a cat’s brain (top).
Husband-and-wife ophthalmologists Joel Pokorny and Vivianne
Smith focus on the retina and a newly discovered ganglion cell
(bottom) that helps the eye adjust to changing light levels.
|
Husband-and-wife team
Joel Pokorny, 63, and Vivianne Smith, 65, have been thinking about
the technology that makes color vision work since meeting as graduate
students at Columbia University. The duo, sitting at face-to-face
desks in an office just around the corner from Shevell’s,
come at their subject from the front end. “We study the retina.
We’re kind of old-fashioned,” says Smith, professor
in ophthalmology & visual science. “It’s OK, we’ve
always been old-fashioned,” retorts Pokorny, a professor in
ophthalmology & visual science and psychology.
Concentrating on the eye may be old-fashioned,
but their findings are not. Their latest research centers on a newly
discovered ganglion cell that helps the eye adjust to changing light
levels. Pokorny and Smith are part of a multi-institutional team
that first reported on the cell at the Association for Research
in Vision and Ophthalmology’s 2003 conference. “When
you walk into a movie late, you first see nothing, then you adapt
and see how to find a seat,” Smith explains. “This ability
ensures good vision at all light levels.” Some retinal diseases
can affect that adaptation, and though such diseases may be linked
to the cell, she says, nothing specific has emerged yet.
Unlike its ganglion counterparts, the MOP cell,
so called because it contains a photopigment believed to be melanopsin,
can signal light’s presence without receiving impulses from
the rods and cones. Textbooks don’t yet cover this “rare
bird,” Pokorny says. Give them a year or two, Smith guesses.
For now, the pair is preparing an article on the cell, which has
been identified in zebra fish, rats, and mice. Although Smith and
Pokorny haven’t decided on an approach, they plan to probe
how it functions.
Other retinal-based projects, meanwhile, including
investigating the 20 or more visual pathways that begin in the retina,
keep them busy. “These pathways,” a research abstract
explains, “send their messages in parallel through the optic
nerve to the brain. In the brain there are a similar number of distinctive
brain structures that receive this retinal alphabet. The nature
of what the eye tells the brain is thus much more complex than expected.”
They want to identify those pathways, from starting point to finish—bringing
them to the brain. “The brain,” Smith concedes, “that
is where there’s a big push.”
Numbers justify the push:
about one-third of the human brain is devoted to some aspect of
seeing, Shevell notes. But much about processing sight remains unknown.
“To me, brain research resembles physics in the early 20th
century,” says Bradley, an assistant professor in psychology.
“People had to get used to concepts,” such as special
relativity and quantum theory, “before they knew what questions
to ask. To really understand the brain, you have to take your imagination
in new directions.”
Imagine, for instance, a device that restores
sight to the blind. Bradley has—and with a multi-institutional
team of scientists, including Robert Erickson, associate professor
in neurosurgery, and Vernon Towle, professor in neurology and surgery,
he’s working on making the concept a reality. Because the
ma-jority of blind patients have damaged eyes or optic nerves, some
researchers try to induce sight by focusing on the eye. But Bradley
and his colleagues have gone straight to the brain. “Most
blind patients could potentially see if you stimulate the brain
directly,” he argues. “The brain’s language is
electrical pulses.”
To arrive at a human-ready prosthesis—Bradley
hopes within the next five to ten years—the scientists must
clear both technological and biological hurdles. As the Illinois
Institute of Technology, a collaborator on the project, explains
on its Web site, success “depends upon providing the cortex
with a well-controlled...stimulation pattern that mimics the pattern
of neural activity normally associated with vision.” Providing
that pattern represents a major challenge because “it is likely
that a large number, at least hundreds, of parallel channels of
stimulation are required.”
The team has made electronic and surgical strides,
developing an implantable device including a stimulator, powered
by a wireless link through a computer system, and 100 to 300 microscopic
wires, or electrodes. The quarter-sized circuit board is filled
with computer chips and wire connections. “My job is to figure
out how to communicate with the brain with these things,”
says Bradley, 43. The group is currently testing the technology
with rhesus monkeys, selected for their trainability and humanlike
visual system. “We’re approaching it scientifically,”
he says in his deliberate style, “taking it step-by-step.”
While the visual prosthesis is a work in progress,
another Bradley venture has reached fruition after four years. “That
project was so hard we’re still panting,” he laughs,
pausing to refuel from a Diet Pepsi can. In September 2003 his team,
known as BradLab, recorded a behavioral change in neurons when perception
shifts. Displaying computer-screen images of moving patterns, morphing
from two objects to one and back again, the researchers trained
three rhesus monkeys, using a liquid-reward system, to indicate
what they saw. Implanted electrodes measured their brain activity.
“Neurons pulsate kind of like the Morse
Code,” Bradley explains. When the monkeys perceived a single
moving object, activity condensed in one place. With a pair of objects,
two clusters of activity thrummed. “Knowing what the monkeys
perceived, and knowing how the neurons responded,” he notes
in a research description, “we can begin to piece together
what is referred to as the neural code”—perception’s
road map.
The project, like most of Bradley’s work,
demands dabbling in diverse disciplines, a fact reflected in his
Green Hall office. A developer’s guide to database applications
sits on his desk alongside multiple computers. Formulas decorate
a wall. Math, he notes, is key. “We create models that try
to imitate the behavior of the brain,” he says. “When
you imitate something you learn a lot about it.” For example,
BradLab postdoc Gopathy Purushothaman used a model to prove his
hypothesis suggesting which mechanisms cause neurons to gain sensitivity
when a subject catches on to a task—in this case, a monkey
judging the direction of a moving pattern.
“What’s missing in vision research,”
Bradley contends, “is a mathematics that has a similar function”
as calculus has to astronomy. “Many of us hope that one day
a simple principle will be discovered that explains how such extraordinary”
computation occurs—how the brain encodes a complex world.
In the meantime, without a numerical shortcut to understanding the
visual system, researchers must navigate its myriad paths.
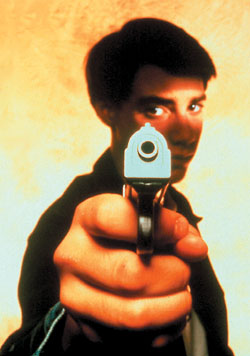
|
Psychologist
Vera Maljkovic believes humans comprehend a scene, like a teenager
with a pointed gun, based on remembered data. |
Naoum Issa, assistant professor
in neurobiology, pharmacology, and physiology, follows those
paths to where the primary visual cortex processes detailed information.
For 36-year-old Issa, working in the trenches is more than a state
of mind. “It’s tough to find my office if you haven’t
already been there,” he warns in an e-mail. Indeed, down in
the Surgery Brain Research Institute’s labyrinthine basement,
his headquarters elude most visitors. He makes a habit of meeting
them at the ground-floor entrance.
Issa takes a similar approach to explaining his
research, delving deeper only after walking through the first level
step-by-step. “To me the coolest thing is understanding how
information is stored in the visual system, how it’s moving
from place to place, and what kind of math is being done at each
step,” he says, ultimately narrowing his focus to “how
the brain limits our ability to see well.”
To lay bare the mechanisms underlying that limitation,
Issa investigates two situations where visual acuity, “a measure
of how small a detail you can see,” suffers. The first, amblyopia,
a dimming of sight in one eye, affects nearly 3 percent of the U.S.
population. Because the disorder “persists even after correcting
physical problems with the eye, it is a pathology of the central
nervous system,” his lab’s Web site explains. “Understanding
how spatial information is disrupted in the amblyopic brain is therefore
central to understanding this problem.” To study what goes
wrong in the primary visual cortex, he shows amblyopic cats a moving
bar on a computer screen, through both their good and bad eyes,
and compares the brain’s response. “We predict that
specific domains of the visual cortex are affected more profoundly
by amblyopia than are other areas.”
The second case involves dynamic acuity, an aspect
of motion perception that shifts as objects speed up or slow down.
“It turns out the eye can follow a fast change in light,”
he says. “The brain can’t. It throws away that information.
Presumably it’s doing that for a reason.” For example,
“if you look at a stationary train, you can see all the detail,”
he notes. “But when the train’s moving quickly, it blurs.”
As a postdoc at the University of California,
San Francisco, Issa mapped where a cat’s brain represents
such “big” (the train) and “very fine” (the
detailed exterior) information, called low and high spatial frequencies,
reporting his findings in the November 2000 Journal of Neuroscience.
Expanding that work, he has returned to cats watching a moving bar,
this time at different speeds, recording their neural activity.
The data indicate that as speed goes up, activity
increases in the low spatial frequency domain and decreases in the
high. Bingo. He has his subregions—in the primary visual cortex’s
frontmost section. “Nobody knew where it happened. We’re
showing it now,” he exclaims in an April phone call. Pop music
plays in the background. Next up: determining “what properties
of the brain cause that to happen.”
With results in hand, Issa takes a step back.
“The real goal is to understand how images are represented
in the brain,” he says. “The field has done a good job
of representing how the brain represents a static image. The next
challenge is how the brain deals with changing images.”
When the conversation turns
to changing images, memory inevitably comes up. Enter Vera
Maljkovic, assistant professor in psychology, for whom something
remembered is a professional calling. “I was always on the
verge between the visual stuff and higher-level things like memory,”
Maljkovic, 41, says from Green Hall’s Visual Perception Laboratory.
“Memory is extraordinarily complex because it’s any
change in the mind that is the result of some experience. It’s
just this enormous amount of information we store about everything.”
Narrowing her scope, she investigates visual
memory—“matching aspects of what you see to the information
in your head”—in the short-term, a matter of mere seconds.
Her studies explore the steps people take to understand their surroundings
and to make decisions. “The entire process depends on memory,”
she explains. “You cannot really comprehend anything unless
you have a memory to begin with. You have to have a knowledge base
already in your mind.”
People rely on such knowledge when faced with
competing visual stimuli as they execute saccades—the quick
eye movements “we use most of the time to look around,”
as the lab’s Web site puts it. To receive data, humans turn
either their attention—the brain’s focus—or their
gaze toward what interests them. “The two behaviors are tightly
related,” the Web site explains. But the mind is a step ahead.
“It is in fact attention that shifts to the target first,
thereby directing the eye movement to a particular point in space.”
Attention also has a stake in what gets remembered,
according to Maljkovic’s latest research. “The things
that go into memory are those things we attend to, that attract
our attention,” she says, breaking down the connection: “Every
time you see something, if you pay attention, it leaves a memory
trace.” Testing the mechanism, she timed how long it took
human subjects to identify the irregular shape in a trio. As patterns
repeated, the responses got faster and faster. The memory trace
helps “us know where to look next,” she says. “You
can pay attention to relevant things and you’re faster.”
In another experiment on “things happening
quickly over time,” Maljkovic moves into muddier territory:
comprehending a scene. “Picture memory is far more complex
because there are many objects, and you have to recognize their
relationships and understand some emotional significance within
those relationships,” she notes. Yet “people can comprehend
scenes in a remarkably short amount of time.”
To measure that ability, she showed subjects
a series of 384 color photographs at different durations, from 13
milliseconds to two seconds per image, and recorded how fast they
grasped a positive, negative, or neutral meaning. The photos included
a man swigging liquor from the bottle, an astronaut floating in
outer space, a teenager with a pointed gun, and a woman nursing
an infant. Subjects correctly categorized more than 20 percent of
the images at the shortest intervals, and with additional time the
percentage increased. At 200 milliseconds they had near-perfect
scores. Such proficiency demonstrates, Maljkovic concludes, that
“we understand the emotional content of an image within a
single glance.”
Teaming with Dartmouth computer scientist Hany
Farid, she took her finding a step further. Farid constructed a
computational model that categorized several of the positive and
negative photographs by contrast, orientation, and spatial frequency.
Using that data, he then asked the computer to predict an unfamiliar
photo’s meaning. The computer generally guessed wrong, suggesting
that the subjects needed more than purely visual clues to understand
a scene. It “seems that people comprehend the image based
on memory,” she says, “and then they decide its meaning.”
It’s another complex function of visual
perception yet to be understood. Maljkovic’s attempts at quantifying
memory dot a white board in her office—models and formulas
that suggest seeing is a numbers as well as a mind game. “We
don’t think vision is hard,” she admits, “because
we do it. So what’s the big deal? It is easy, but the machinery
is so complex.” Along with Chicago’s other visual perception
researchers, she’s trying to describe the machinery in a way
that make seeing as simple as it looks.
Seeing
is believing, right?
Not when the mind plays TRICKS, as
with chromatic induction from grouping.
Color is a mental, not physical, phenomenon.
Red, orange, yellow, green, blue, indigo, violet: such hues
represent the brain’s responses to light of varying
wavelengths. As a result, things aren’t always what
they seem.
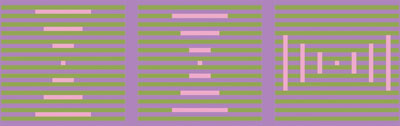
Consider the images above. Composed of
rectangular bars, the three hourglasses look different because
of their placement within patterned backgrounds of purple
and green stripes. It’s another case of chromatic induction—shifts
in color appearance caused by nearby light, or an object’s
context.
But there’s more. Check out the center
squares. Each one is printed on a purple stripe with green
directly above and below. Yet even with the same surrounding
light, the squares appear multiple shades, echoing the larger
hourglass shapes.
That’s grouping,
says Steven Shevell, a professor in psychology and ophthalmology
& visual science. “Fragments of light that belong
to the same object are grouped together to be perceived as
a whole.”—M.L.
|
|
|