INVESTIGATIONS
Code reader
After revolutionizing methods of studying
genetics, Wen-Hsiung Li reads deep into DNA sequences to unravel
molecular mysteries.
At 61, Wen-Hsiung Li thought he was too
young to win a Balzan Prize, often referred to as the Italian Nobel.
The prize, given to four winners each year, two in scientific disciplines
and two in humanities, had been awarded previously in Li’s
field, genetics and evolution, but only to octo- and nonagenarians.
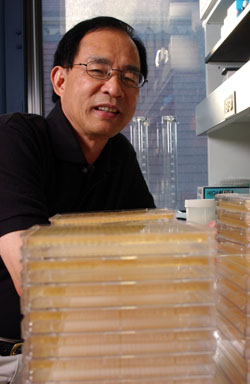
Photo by Dan Dry |
Molecular biologist Wen-Hsiung Li’s
lab grows stacks of yeast. |
Yet in September the Milan–based International
Balzan Foundation named him a winner, and in November Li, the George
Beadle distinguished service professor in Ecology & Evolution
and the Committee on Evolutionary Biology, traveled to Berne, Switzerland,
to accept his award. The foundation cited Li’s “many
fundamental contributions to molecular evolution,” including
developing methods “for inferring evolutionary relationships
from comparison of DNA sequences” and for “estimating
the degree of accuracy of evolutionary trees.”
Molecular evolution has fascinated the Taiwan
native since the late 1960s. As a Brown University doctoral student
in applied mathematics, he noticed that biology was one branch of
science “in which mathematics was not so well developed,”
and that applying math to genetics in particular might help to better
explain many “mysteries in life,” such as why children
resemble their parents.
Before joining Chicago’s faculty in 1999,
Li, who wrote the first textbook on molecular evolution, spent 26
years at the University of Texas Health Science Center in Houston.
As a postdoc there he began research on the “molecular clock,”
or the rate at which species’ genes mutate, determining their
genetic diversification. That work produced one of his first major
contributions to the field.
Since 1965 researchers had used protein sequences
to study molecular clocks, theorizing that protein sequences evolved
at a constant rate across species. By the 1980s data for DNA sequences—which
contain more information than protein sequences—had become
widely available, and Li realized that they could better determine
gene-mutation rates. In 1985 he and Chung-I Wu, also a University
of Texas postdoc and now chair of Chicago’s Ecology &
Evolution department, employed DNA data to show that molecular clocks
actually are dependent on generation time: the shorter a species’
life span, the faster the clock. A rodent’s molecular clock,
for example, advances five times faster than that of humans.
Even more important than their discovery was
the method by which they found it. Comparing human and rodent DNA
sequences revealed that the two were different, but not which species
evolved faster. So Li brought in a third organism, called an out-group,
equally related to both human and rodent by time elapsed since splitting
from a common ancestor—in this case a dog, cow, or rabbit,
depending on which genes were available. Li could tell whether the
rodent or the human had evolved faster by charting which animal’s
DNA sequence varied more from the out-group’s. A computer
analysis showed that the rodent’s sequence had changed more
than the human’s—so the rodent had evolved faster. This
“relative rate test,” using a third organism equally
related in evolutionary time to both species under question, is
now a standard method for molecular biology research.
In another major contribution, Li improved ways
to test statistical confidence in evolutionary trees—charts
tracing organisms’ genetic relationships, such as the 1980s
tree demonstrating that humans and chimpanzees are more closely
related than chimps and gorillas. In 1996 he created a method of
randomly selecting genetic coding from different nucleotide sites
to compare organisms. Like a statistically accurate survey using
a random sample, the more often the two organisms appear to be similar,
the higher the confidence that the evolutionary tree is accurate.
With the recent flood of biological data from
the Human Genome Project, Li’s research scope has broadened
dramatically. “In the old days we looked for genes by disease
or by trait,” he says. Now biologists study specific DNA sequences
and try to figure out what they do.
One study that Li says would have taken much
longer before the human genome was mapped involved the genetic mutation
rates in males and females. Mutations occur during cell division,
and because sperm cells divide constantly—as opposed to egg
cells, which are mostly formed when a female is born—there
is increased opportunity for genetic error—and thus evolution—in
males. Two previous studies had argued that the male-female mutation
ratio was only two to one—a difference not large enough, Li
says, to account for human evolution. He and research associate
Kateryna Makova compared human and monkey genetic-sequence data
found on both the Y and X chromosomes, and later on both the Y and
an autosome, or nonsex chromosome, finding a six-to-one ratio, which
they published in the August 11, 2002, Nature. They further
showed that the mutation rate is higher in rodents than in primates—confirming
Li’s molecular-clock research.
The human-genome information also has helped
Li to home in on the mysteries of DNA itself, studying its noncoding
regions—the spaces between the coding—called “junk
DNA” until scientists showed that at least some of it has
specific functions. Li did a computational analysis, reported in
the February 12, 2001, Nature, showing that such regions
make up 46 percent of the human genome. He speculated that noncoding
regions may provide clues to how human genes evolve.
In the coding regions, meanwhile, Li and his
lab colleagues are attempting to unravel the enigma of duplicate
genes. Why, researchers have wondered, would an organism need two
genes with the same coding? In two articles this year—one
in Trends in Genetics and one in Genome Research—Li
has begun to explain this apparent redundancy. His lab, using microarrays
that brighten as more copies of a gene are expressed, showed that
in both yeast and humans, after genes duplicate, their expression
patterns—when and where they are activated—change rapidly.
So both genes, if used in different times and places, may be necessary.
Li’s lab is studying why those expression
patterns might alter. Second-year graduate student Geoffrey Morris,
for example, is investigating yeast DNA’s noncoding regions,
which, he says, control gene expression. In another experiment Li
and Zhenglong Gu, PhD’03, removed each of the 6,000 genes
in a yeast sample, one by one, to test how each gene’s removal
would affect the yeast’s growth. As they reported in the January
2, 2003, Nature, the yeast grew better if the removed gene
had a duplicate inside the sample than if the gene was unique. Duplicate
genes, they showed, may help protect against harmful mutations.
The findings emerging from Li’s lab at
such a rapid pace may come to show that his earlier work, which
helped him win the Balzan Prize, was only the beginning of a prolific
career. Reading deep into DNA’s nether regions could yield
an important encore.—A.M.B.
|
|